A research team led by YongKeun Park at KAIST Institute for Health Science and Technology has introduced a novel computational adaptive optics (AO) approach to enhance thick tissue imaging. The study, published in Nature Communications, presents a digital aberration correction technique that exploits the tilt-tilt correlation from the optical memory effect, significantly improving the quality of deep tissue imaging. By detecting and compensating for optical aberrations in a transmission-mode holotomography setup, the proposed method enables accurate visualization of three-dimensional biological structures in thick human tissue samples.
Traditional AO methods, including wavefront shaping and guide-star-based techniques, have faced challenges in biological tissue imaging due to scattering-induced aberrations and motion artifacts. Unlike conventional approaches that require physical wavefront modulators, this computational method detects phase differences in aberrated wavefronts using small tilts in incident waves. The researchers developed an aberration matrix that characterizes the optical memory effect, allowing for robust aberration correction even in highly scattering and moving samples. Their experimental results demonstrate substantial improvements in imaging thick tissues, with significant restoration of fine biological structures that were previously obscured by aberrations.
The study validates the effectiveness of this method through experiments on human pancreatic and intestinal tissues, with sample thicknesses ranging from 10 μm to 100 μm. The results show that the proposed approach successfully corrects aberrations in transmission-mode imaging, achieving nearly identical image quality to polymer-free reference samples. Moreover, the method proves effective even under dynamic conditions, as demonstrated in the correction of moving silica bead samples. The researchers further highlight the scalability of this technique to complex in vivo imaging applications by simulating its performance in time-gated reflection microscopy.
By leveraging the angular memory effect and computational AO, this work provides a breakthrough in high-resolution deep tissue imaging without the need for invasive modifications or additional optical elements. The proposed method is expected to have significant implications in biomedical imaging, particularly for histopathology, neuroscience, and drug discovery, where accurate visualization of thick biological samples is critical. Future research aims to extend this approach to in vivo imaging and real-time applications in medical diagnostics.
This work was supported by National Research Foundation of Korea grant funded by the Korea government (MSIT) (RS-2024-00442348, 2015R1A3A2066550, RS-2022-NR068141), Institute of Information & communications Technology Planning & Evaluation (IITP; 2021-0-00272) grant funded by the Korea government, the Ministry of Trade, Industry and Energy (MOTIE) and Korea Institute for Advancement of Technology (KIAT) through the International Cooperative R&D program (P0028463), and the Korean Fund for Regenerative Medicine (KFRM) grant funded by the Korea government (the Ministry of Science and ICT and the Ministry of Health & Welfare) (21A0101L1−12).
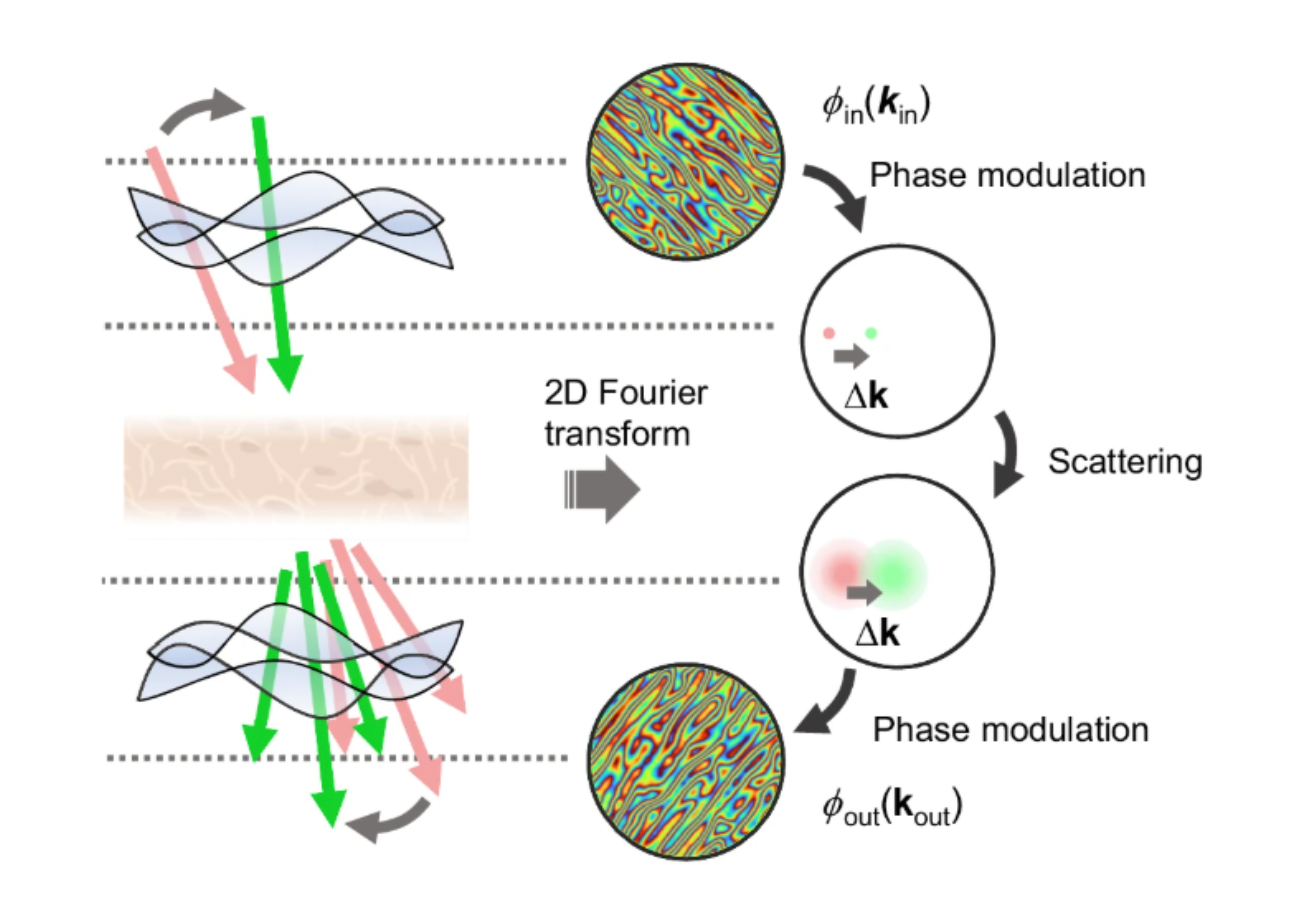
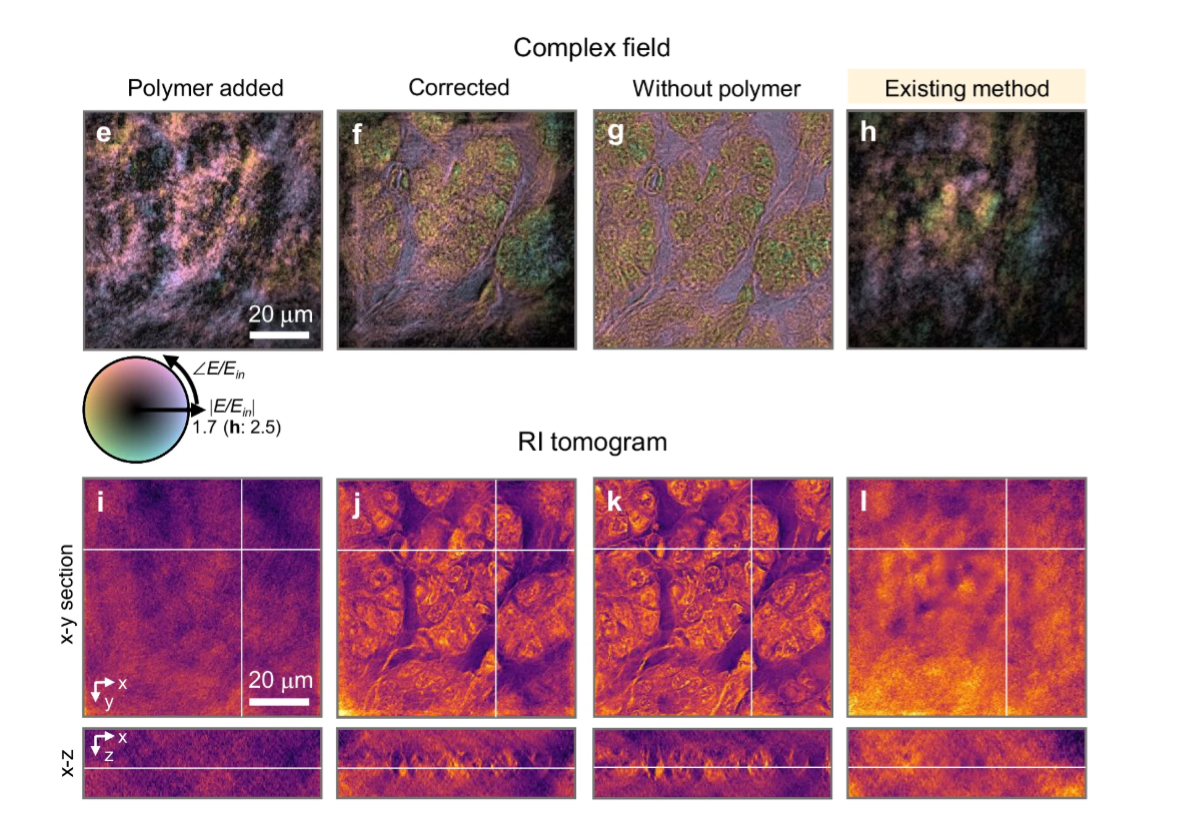
ChulMin Oh, Herve Hugonnet, Moosung Lee, YongKeun Park Department of Physics
E-mail: yk.park@kaist.ac.kr
Homepage: https://bmokaist.wordpress.com