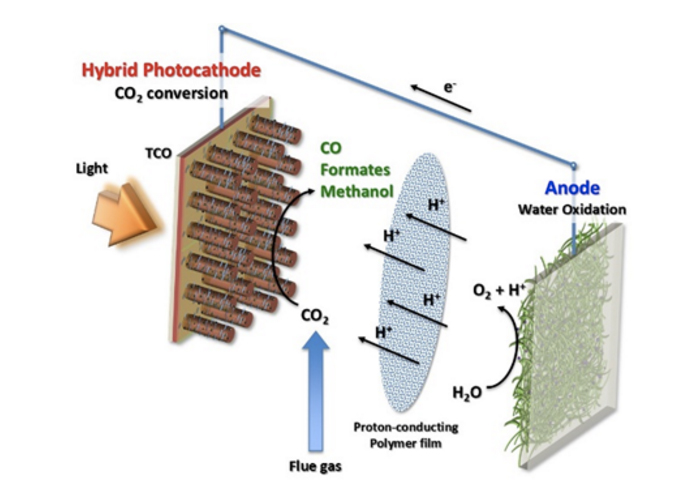
Plants harness energy from sunlight to make hydrocarbon energy sources via reaction of carbon dioxide (CO2) and water (H2O). The reaction, known as natural photosynthesis, has low solar-to-fuel efficiency of less than 1%. Evolutionary optimization has embraced low efficiency, which is sufficient to keep the plant population on earth.
Consumption of fossil fuels by humans with increasing energy demands has triggered the rapid increase of CO2 emission. Converting CO2 to hydrocarbon, just like plants do, has been considered an alternative way to mitigate CO2 emission and ultimately meet energy demand. While the plants and the ecological systems can accommodate the low efficiency of the natural photosynthesis, it is inadequate to meet the current demands of human energy consumption. A solution for the pressing energy and environment problems would require a high-efficiency process.
In many of the previous approaches, energy is required for the chemical reduction of CO2. As a result, net CO2 amount will go up as conventional ways of generating energy such as electricity or thermal energy leads to the generation of CO2. The photoelectrochemical (PEC) approach utilizes two electrodes, on which reduction and oxidation take place, and solar energy is absorbed by the electrodes to facilitate the production of hydrocarbon fuels from CO2 and water as can be seen in the figure. The PEC cells have been considered promising since solar photons provide clean energy that can help the reaction run uphill in terms of energy and good catalysts, which would alleviate the kinetic barrier.
To achieve fabrication of an efficient photoelectrode, many researchers have tested various structures of electrodes using organic or inorganic materials. Organic semiconductors have attracted remarkable interest due to its low weight and manufacturing cost. However, electron-hole pairs in organic materials exhibit a strong binding energy, which makes it difficult to dissociate an electron and a hole. In contrast, inorganic semiconductor electrodes can be efficient because little energy is needed to dissociate excitons into charge carriers.
Once the electrons and holes are separated to a cathode and an anode, they react easily in reduction and oxidation, respectively. With this advantage, inorganic semiconductors have been employed electrodes, yet there have been marginal improvements in CO2 conversion efficiency. In PEC cells designed for CO2 conversion, a fundamental yet often overlooked issue is transferring multiple photo-excited electrons to the catalyst particles. For example, TiO2, a typical photoanode material, exhibits low light absorption (bandgap > 3.2 eV) and poor photo-carrier collection, leading to negligible solar fuel generation efficiency (< 0.1%). Therefore, photoelectrodes that can convert CO2 efficiently has to be developed.
Development in photoelectrodes that can convert CO2 efficiently
To develop a highly active CO2 conversion photocathode, an intramural collaborative research team led by Professor Doh Chang Lee at the Saudi Aramco-KAIST CO2 Management Center (Co-PIs: Professor Yoon Sung Nam in the Department of Materials Science and Engineering and Professor Hyunjoo Lee in the Department of Chemical and Biomolecular Engineering at Korea Advanced Institute of Science and Technology) has employed a multi-component hybrid system: silicon nanowires – oxide shell passivation– molecular catalysts. Silicon, which has a relatively lower band gap (1.12 eV), allows the photocathode to produce enough photocurrent enabling multi-electron transfer. However, Si reflects more than 25% of sunlight striking its surface. To address this challenge, the research team proposed to develop a defect-free Si nanowire array for an efficient CO2 reduction. This Si nanowire photocathode platform provides increased surface area for additional tethering sites and thus further lowers the overpotential. In addition, core-shell junction provides additional photovoltage up to 600 mV for CO2 reduction.
This research also addresses a stability issue of the electrode. The surface of Si is easily oxidized into SiO2, which prevents electrons from participating in reduction. A thin TiO2 layer on the Si nanowires can protect the Si surface from photocorrosion. This TiO2 layer is chemically inert for a wide range of pH solution. In addition, the conduction band edge of TiO2 is located lower than that of Si, so that photo-generated electrons in Si can be injected into Si to reduce CO2.
Carbon dioxide conversion can produce a few different byproducts of hydrocarbon molecules: CO, formic acid, formaldehyde, methanol, or methane by reduction. For the selective conversion of carbon dioxide into formic acid, new types of Ru-based molecular catalysts is prepared and immobilized on Si nanowire based photocathode. This new type of hybrid photocathode will have solid support such as silicon or TiO2 as the electrode, and molecular catalysts are deployed on them. The electrode can harvest visible light, and induced electrons are transferred into the core metal of molecular catalyst. Not only the selectivity within the products of conversion but also selectivity against the competing hydrogen evolution will be addressed.
Promise in future CO2 conversion photocathode
This work focuses on the design of photoactive cathode in a PEC cell that increases the reaction rate for carbon dioxide conversion. It is expected that the hybrid photocathode will outperform the quantum efficiency of natural photosynthesis. Immobilization of Ru-based catalysts on a photocathode has been attempted for the first time, different from previous studies that focus only on decrease in overpotential. The deposition of Ru catalysts will enhance the efficiency to 3% with increased light harvesting of Si nanowires. Few reports on carbon dioxide conversion have demonstrated the conversion yield to be remotely close to a commercially meaningful scale. This work will be a seminal starting point to open the possibility of maximally utilizing light-matter interaction in PEC cells.